Life Cycle Analysis of hydrogen production with PEM electrolysers
Published on 19 March 2022
Polymer Electrolyte Membrane electrolysers (PEM) are low temperature (<100 °C) electrolysers using a solid membrane as separator between the anode and the cathode. They have approximately the same efficiency as alkaline electrolysers (AEL) but operate on a larger current (about 5 times higher than AEL), so that they are significantly more compact for the same hydrogen delivery. PEM are also more tolerant to short-term load variation and therefore more appropriate for a coupling with variable renewable resources.
Yet, despite all these advantages, the commercial availability of PEM electrolysers has not led to the disappearance of alkaline electrolysers. The key reason is the reliance of PEM electrolysers on very specific and expensive materials. Beyond the economic aspect, this reliance also implies a larger environmental impact, especially on use of mineral resources.
Materials
The acid environment related to H+ transport imposes the use of iridium and platinum for the anode and cathode, respectively. Because these are expensive and difficult-to-source materials, they are only used as coating or extremely thin layers over a core material.
Titanium is the core material for electrodes but also for the interconnect, i.e. any of the internal electricity conducting component. Accordingly, a 1-MW PEM electrolyser is made of over half a ton of titanium but less than 1 kg of iridium or platinum.
Such metals are more difficult to source than steel or aluminium and have a larger environmental impact. This explains why the material sourcing and manufacturing of PEM electrolysers has a higher equivalent GHG impact than the material sourcing and manufacturing of alkaline electrolysers. For an identical surface of reaction, the PEM electrolyser has a 16-time higher GHG impact that the AEL electrolyser (stack only). But productivity is also a lot higher thanks to the higher current (about 5 times higher), so that the ratio in equivalent GHG emissions per kg of H2 produced is below 4.
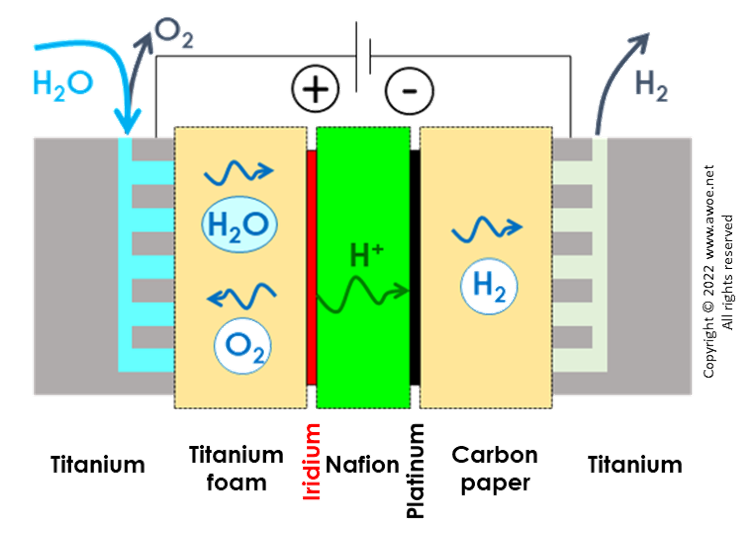
Key materials in the stack of a PEM electrolyser
The oxygen electrode (anode) accounts for nearly half of the GHG impact because of its composition of iridium oxide over titanium core. The rest of the interconnect made of titanium represents another 35% and the hydrogen electrode made of platinum over titanium lies between 10 and 15%. These 3 components define 98-99% of the GHG impact of the PEM stack.
Balance of Plant
In addition to the stack of cells, an electrolyser installation also includes pumps to circulate water (from electrolysis and for cooling), purifiers (for water and for hydrogen) as well as electric connections (transformer and rectifier) and some power and control electronics.
All these components require significant amounts of materials, mostly metals. Furthermore, the electrolyser and its auxiliary are hosted in a building, adding some concrete for the foundations and at least a light structure to protect sensitive equipment from bad weather.
Most of these devices are made of steel or aluminium, with the exception of foundations made of concrete. Accordingly, a functional PEM installation contains significant amounts of steel, aluminium and concrete, even if there are none in the electrolyser itself. The electric components (transformer, rectifier) also add copper to the list of key materials.
On a per weight basis, the highest share is for construction elements (~80% for foundations and building), followed by the auxiliaries and finally only about 5% for the core of the electrolyser.
Efficiency
Electrolyser operation has water and electricity as main inputs. While about 9L of water is required per kg of produced hydrogen, there is an even larger water consumption for cooling (about 10 times). The GHG impact of water is low but the water treatment ahead of usage in the electrolyser adds to the electric consumption of the installation.
With an efficiency of ~65%, the electric consumption is ~60 kWh/kg H2. This large consumption implies that electricity is the main contributor to the overall GHG impact of electrolyser operation. With clean electricity from the best wind turbines, electricity carbon intensity is 7 gCO2,eq/kWh leading to a GHG impact of hydrogen of 420 gCO2,eq/kg H2 due to the electricity consumption only.
Life Cycle Analysis
Assuming a life cycle of 20 years and operation of 8000 h/year, the stack components of a PEM electrolyser will certainly require replacement, which means replacing the expensive electrode with iridium or platinum plating. As the lifetime of PEM electrolysers is at best 50,000 h, 3 stack replacements are a minimum, largely increasing the amount of consumed platinum-group metals.
Published figures on carbon intensity for the electrolysis stack shows about 200 to 300 gCO2,eq/kg H2 once accounting for stack replacement for long hours operation over 20 years. There are an additional ~10 gCO2,eq/kg H2 for the balance of plant and ~4 gCO2,eq/kg H2 for water consumption. Although non zero, these numbers remain below the impact of electricity consumption.
With a total electricity consumption (stack+auxiliaries) of 60 to 65 kWh/kg H2, the carbon intensity of electricity has a very large influence on the environmental impact of the produced hydrogen. Considering the cleanest electricity from wind turbines, the impact of electricity consumption can be as low as 420 gCO2,eq/kg H2 leading to a total impact of hydrogen as low as 620 gCO2,eq/kg H2. With a renewable mix combining various types of wind farms and some photovoltaic panels (electricity carbon intensity is 42 gCO2,eq/kWh, the impact of electricity increases up to 2700 gCO2,eq/kg H2, so that the stack impact becomes secondary (<10%).
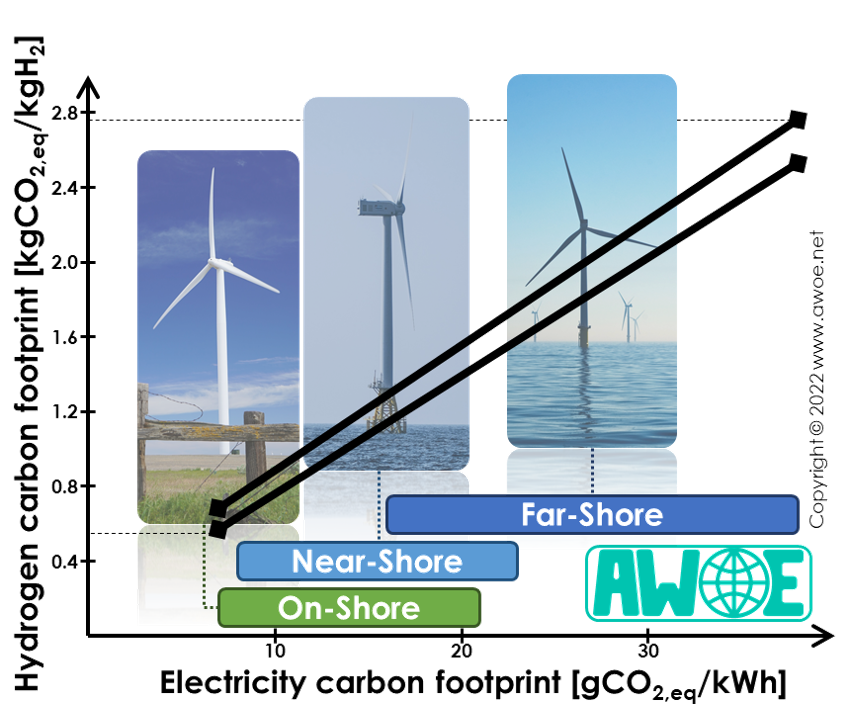
Carbon footprint of green hydrogen derived from wind turbine electricity with a PEM electrolyser